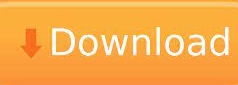
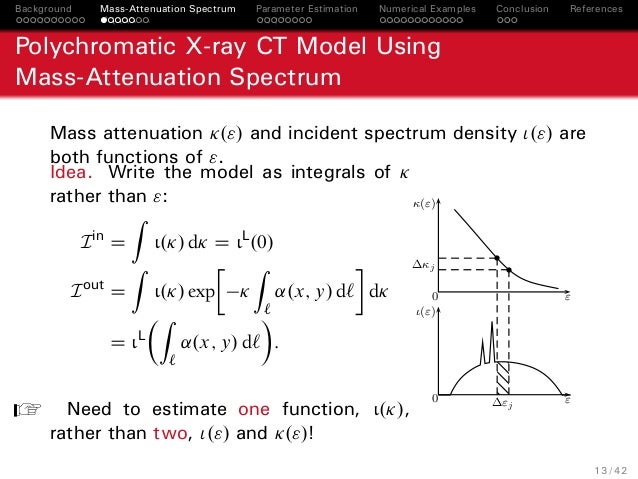
In the same way, X-rays lose their energy, or "harden", more quickly as they pass through metal or bone than as they pass through muscles or organs. And if I’m swimming through water, I expend less energy than if I were swimming through, say, Jell-o. As I swim, I lose energy as I cross the pool I’m more tired the further I go. Here's an analogy to help understand beam hardening: P retend I’m swimming across a pool. I am an X-ray beam and the pool is the part of the body being scanned. See an example of an artifact caused by beam hardening below. Bright streaks are seen adjacent to the dark streaks 2. These phenomena produce dark streaks between metal, bone, iodinated contrast, barium, and other high-attenuation materials. Most streak artifacts occur near materials such as metal or bone, primarily as a result of beam hardening and scatter.

The name should suffice to tell you what a streak artifact looks like, but the causes bear some explanation. In spite of this, CT is commonly susceptible to a number of image anomalies 1, including streak artifacts. The technology is a mainstay in the imaging stables of most hospitals.
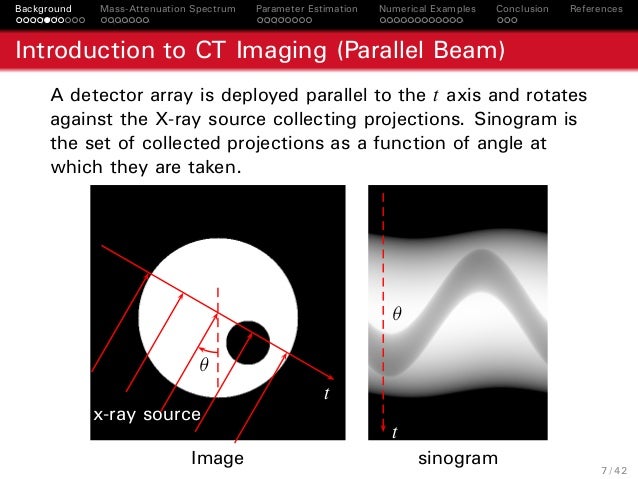
arterial spin labeling (ASL) MR perfusion.dynamic contrast enhanced (DCE) MR perfusion.
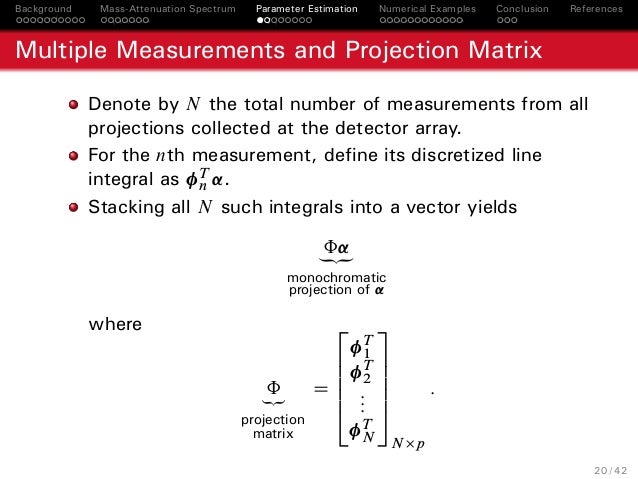
dynamic susceptibility contrast (DSC) MR perfusion.metal artifact reduction sequence (MARS).turbo inversion recovery magnitude (TIRM).fluid attenuation inversion recovery (FLAIR).diffusion tensor imaging and fiber tractography.MRI pulse sequences ( basics | abbreviations | parameters).iodinated contrast-induced thyrotoxicosis.iodinated contrast media adverse reactions.clinical applications of dual-energy CT.as low as reasonably achievable (ALARA).Many modern scanners are now also equipped with metal artifact reduction algorithms that utilize iterative reconstruction to limit beam hardening artifacts 3. Streak artifacts can sometimes effectively be reduced by increasing tube voltage (better penetration of high-density objects), or by using a dual-energy imaging approach. Often an attenuating substance (usually metallic) is appropriated to harden the beam before it reaches the patient.ĬT scanners often need to be calibrated with vendor-specific phantoms to overcome unavoidable beam hardening artifacts such as cupping. Most modern CT scanners utilize filters in an attempt to overcome beam hardening. The characteristic "cupped shaped profile" of the CT numbers is best demonstrated when scanning phantoms 1,2. Since simple beam hardening correction is built into modern scanners, cupping artifact is not usually encountered during clinical imaging. If uncorrected during CT reconstruction, these differences in the expected attenuation profile lead to a perceived peripheral dense appearance. As the beam becomes harder and passes a higher mean beam energy, the lower attenuation coefficient means the CT number goes down for longer paths. Cupping artifactīeam hardening will cause the middle of the image to decrease in value, not increase edge value, as the lower energy photons preferentially get attenuated over longer path lengths. It is the result of the polychromatic x-ray being ‘hardened’ at different rates according to rotational position of the tube/detector. Streaking may also occur along the long axis of a single high attenuation object. Streaking artifact appears as multiple dark streaking bands positioned between two dense objects, for example at the posterior fossa. CT beam hardening artifact has two distinct manifestations, streaking (dark bands) and cupping artifacts. bone or iodinated contrast) may result in characteristic artifacts. In CT, beam hardening from a very dense target (e.g. This same phenomenon is exploited in radiography and CT, by use of metal filters in order to "pre-harden" the x-ray spectrum and minimize low energy photons (see filters) 2. The effect is conceptually similar to a high-pass filter, in that only higher energy photons are left to contribute to the beam and thus the mean beam energy is increased ("hardened") 1. Beam hardening is the phenomenon that occurs when an x-ray beam comprised of polychromatic energies passes through an object, resulting in selective attenuation of lower energy photons.
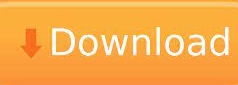